The latest version of a filter
synthesis program for the personal computer supports the creation of microstrip,
stripline, lumped-element, and waveguide filters.
Filter designers have a wide choice of commercial software design tools at their
disposal.But the latest version (Version 3.0) of the MMICAD filter synthesis
software suite from Optotek Ltd. (Kanata, Ontario, Canada) now includes userdefined
physical surroundings as an integral part of the synthesis operation. The software
synthesis tool is actually a set of 35 dedicated programs for the design of
microstrip, lumped element, stripline, and waveguide filters. The design interface
and common menu format seamlessly integrates with the MMICAD linear simulator
and physical layout in a user-friendly design environment. A design example
of a parallel coupled bandpass filter will be illustrated in this article.
The parallel coupled-line bandpass filter is one of the more popular microwave-filter
designs. It is suitable for implementation in microstrip and surface-mount
construction. However, there are practical limitations to its design. Design
bandwidths are limited to about 20 or 30 percent around the center frequency
due to decreasing input
coupling gap that is a function of increasing bandwidth and decreasing passband
ripple. There are also practical considerations in construction that includes
the width of the housing, which should be kept less than onehalf wavelength
of the highest frequency in order to suppress the possibility of the TE01 waveguide
mode propagation
that can propagate energy around the filter
1. This dialog box is used for entering the electrical parameters
for the example filter design.
and limit the attainable stopband
attenuation. The presence of a cover over the circuit can cause the passband
frequency to be shifted, and this should be compensated for in the design synthesis.
At higher frequencies, microstrip dispersion must be accounted for if the passband
is to be centered on the design frequency. Other circuit discontinuities, such
as microstrip metalizaztion, and the capacitance at the ends of the coupled
resonator lines, must also be included in the filter synthesis.
2. This data window shows the calculated
even- and odd-mode resonator
impedances for the example filter.
All of these
factors should be included in the synthesis if multiple fabrication trials
are to be minimized and possibly eliminated. The interface to the synthesis
software, the menu system, is organized and arranged to match the sequence
of calculations a designer would normally follow. This gives the designer access
to the intermediate steps in the calculations, and allows the designer the
opportunity to control, interact, and optimize a design during the calculation
process. For example, an electrical design may have been arrived at, and
the microstrip dimensions can then be separately calculated and optimized by
the designer varying the substrate dielectric constant and thickness, the metal
thickness, the cover height, and the type of input coupling. The requirement
is for a filter that operates from 10.0 to 13.0 GHz with greater than 30-dB
rejection at 9.0 and 14.0 GHz will be used as a design example.
3. Microstrip physical parameters for
the filter example are entered into this
dialog box.
4. This data window shows the calculated microstrip dimensions
for the example filter design.
A filter with
eight resonators and a passband ripple of 0.1 dB (return loss of 16.3 dB) will
meet this requirement. The design bandwidth is set to 3.5 GHz centered at 11.5
GHz, which is an F1 of 9.75 GHz and F2 of 13.25 GHz, representing a fractional
bandwidth of 30 percent. Figure 1 shows the dialog window for entering electrical
information for a desired filter, including the number of resonators, passband
ripple, center frequency, bandwidth, rejection frequencies, and impedance.
Figure 2 shows the resulting calculated even- and odd-mode impedances for this
parallel-coupled-line filter design. The synthesis software can also calculate
the filter frequency response, including dissipative losses. The response calculation
can be used to verify that the basic electrical design will meet a set of requirements,
including the increased passband insertion loss at the band edges due to dissipative
losses.
5. This dialog box shows the layout dimensions for the example
filter design, along with the calculated waveguide cutoff
frequency.
|
The calculated response for this design will be generated by Version
3.0 of the MMICAD linear circuit analysis program.5 Figure 3 shows the dialog box for entering the physical parameters
of substrate dielectric constant and thickness, metal thickness, and the type
of input coupling.
For this particular filter design, the substrate is 0.015-in.-thick
alumina with 0.125-mil-thick thin-film metalization. The unloaded quality factor
(Q) for this substrate obtained though experimentis about 225 at X-band.
Because of the tight input coupling required for this large bandwidth, interdigitated
coupling lines were chosen for this filter. The filter’s calculated dimensions
are shown in Fig. 4. For noninterdigitated lines, the line width would be 11.1
mils although the line spacing would be only 0.4 mils, somewhat less practical
to fabricate compared to the interdigitated design. In Fig. 5, a dialog box is
used for specifying the circuit layout and the circuit mask. There is an intermediate
window (not shown) that allows choosing the arrangement of the circuit input
and output lines. Here, input and output lines are selected on a common centerline.This
produces the narrowest layout and substrate width, and maximizes the TE01 mode
cutoff frequency for a metal enclosure. The calculated
cutoff frequency is part of the dialog box and is 33.723 GHz for a substrate
width of 175 mils. This width is chosen for mechanical packaging purposes. The
overall length of the substrate is 1500 mils. Once all required values have been
entered into the dialog box, the software yields a circuit layout that can
be written to a DXF file for direct generation of the photo mask. A photograph
of the filter fabricated from this synthesized file is shown in Fig. 6. The
filter-synthesis program generates a series of netlist files with the calculated
nominal dimensions of the circuit for design verification, as well as files
for analysis of changes in filter response with temperature, files for worst-case
and yield analysis, and netlist files of circuit discontinuities to improve
performance predictions.
6. This layout artwork offers a representation of the fabricated microstrip filter.
Figure 7 gives the filter frequency response calculated
by MMICAD over a wide frequency range. The calculation covers the frequency
range of 6.0 to 20.0 GHz, and it shows the expected rejection of the filter.
Figure 8 gives the calculated response over a narrow band of 9.0 to 14.0 GHz,
and this shows the expected passband insertion loss and return loss frequency
response.
Fabricated Filter
The fabricated filter of Fig. 6 was evaluated with a vector network analyzer.
Measurements of insertion loss, S21 in dB, and return loss, S11 in dB were
saved in a file. This file, in turn, can be included in the MMICAD filter
netlist for direct comparison of the measured and calculated responses.
As a result, Fig. 7 shows that the measured rejection is close to the calculated
performance. The rejection is better than 70 dB, and
is the result of the narrow width of the filter housing suppressing the
propagation of the TE01 waveguide mode. The rejection levels at 9.0 and
14.0 GHz are close to the calculated values. Figure 8 compares the measured
and calculated passband responses. The measured response is close to the
calculated, with the passband centered close to the design. The passband
insertion loss response is also close to the calculated response, although
three consequential conditions should be noted.
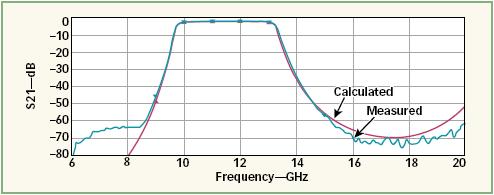
7. The example filter’s measured response compares closely with the response predicted
by the MMICAD software.
First, the pairs of interdigitated
fingers can resonate in a quarter-wave-length transverse-electromagnetic
(TEM) mode and this condition causes a resonance
suck-out in the passband. This was eliminated with bond wires that shorted
the tips of the open circuit ends of the fingers. Second, the test fixture
itself has a known insertion loss of 0.8 dB, due to SMA connector loss
and transition to microstrip loss. Consequently, a 0.8-dB attenuator is
added to the filter circuit netlist to make the calculated losses match
the measured. It is apparent that the measured rounding of the passband,
the result of filter resonator dissipative losses, matches the calculated.
The passband return loss is better than –15 dB, compared to the design
value of –16.3 dB. And third, the measured results are for the first fabrication
of this filter without any tuning or modifications.
The measured data for the fabricated filter is in excellent agreement with
both the design goals and with the MMICAD simulation of the circuit synthesized
dimensions. Only part of the reason for this success is the relatively
tight tolerances that are possible with thin-film alumina microstrip.
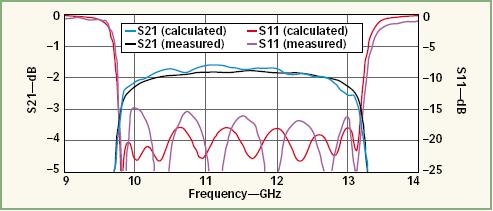
8. The measured insertion loss and return loss for the example filter compares
closely with the responses calculated by the MMICAD software.
The
excellent circuit performance is possible because the synthesis includes
compensation of those circuit details that can alter and degrade the response.
Furthermore, the MMICAD synthesis software facilitates design trade-offs
as part of the synthesis process and it facilitates detailed circuit analysis
by creating the necessary MMICAD netlists.
ACKNOWLEDGMENT
Special thanks to US Microwaves (Santa Clara, CA,
www.usmicrowaves.com) which provided foundry services
for the filter produced for this article.
REFERENCES
1. G. Matthaei, L. Young, and E.M.T. Jones, Microwave Filters,
Impedance-Matching Networks, and Coupling Structures,
McGraw-Hill, New York, 1960, pp. 588-589.
2. M. Kirschning and R. Jansen, “Accurate wide range dynamic
equations for the frequency dependent characteristics of
parallel coupled microstrip lines,” IEEE Transactions on
Microwave Theory & Techniques, Vol. MTT-32, No. 1, January
1984, pp. 83-90.
3. H.A. Wheeler, “Transmission-line properties of a strip on a
dielectric sheet on a plane,” IEEE Transactions on Microwave
Theory & Techniques, Vol. MTT-25, No. 8, August 1977, pp.
631-647.
4. MMICAD SYNTHESIS software, OPTOTEK Ltd., 62 Steacie
Dr., Ottawa, ON K2K 2A9, Canada.
5. MMICAD linear circuit simulation, OPTOTEK Ltd, 62 Steacie
Dr., Ottawa, ON K2K 2A9, Canada.
This has been published in Microwave and RF , Feb 2003.
|